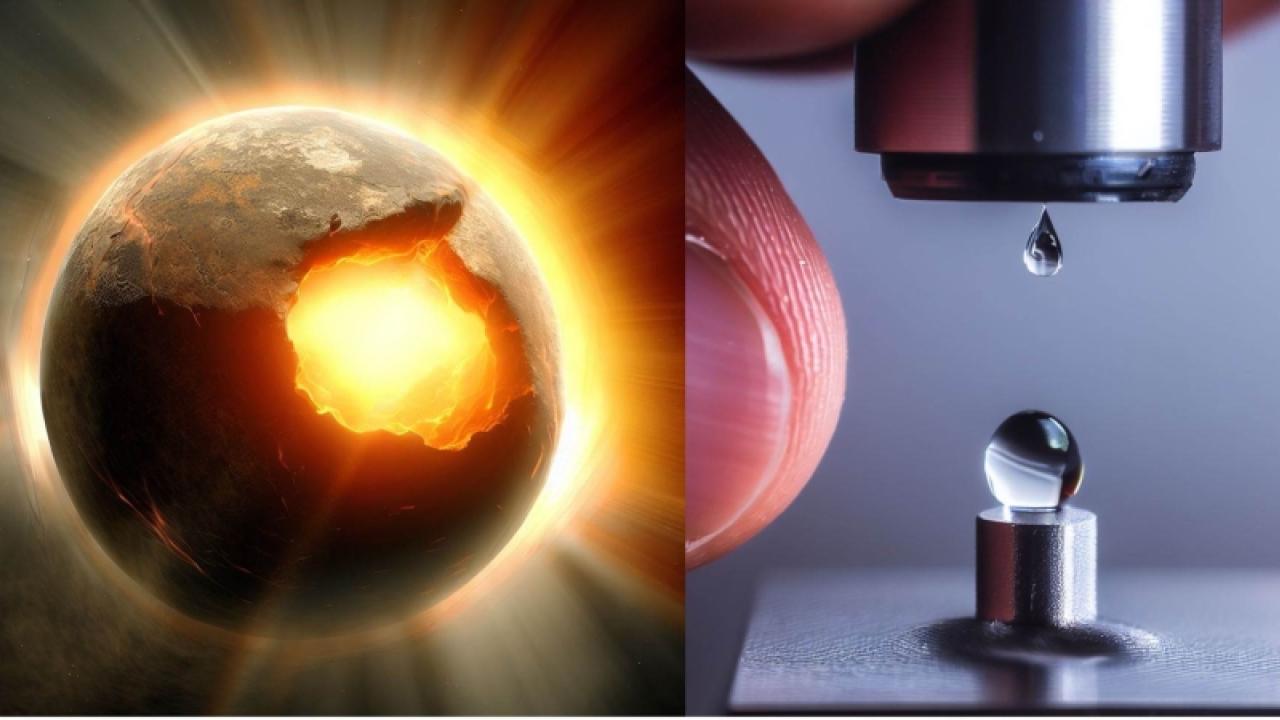
Matter at Extremes: A Question of Scale
Mechanical and Aerospace Engineering Professor Jean-Pierre Delplanque develops new scaling law with two national laboratories
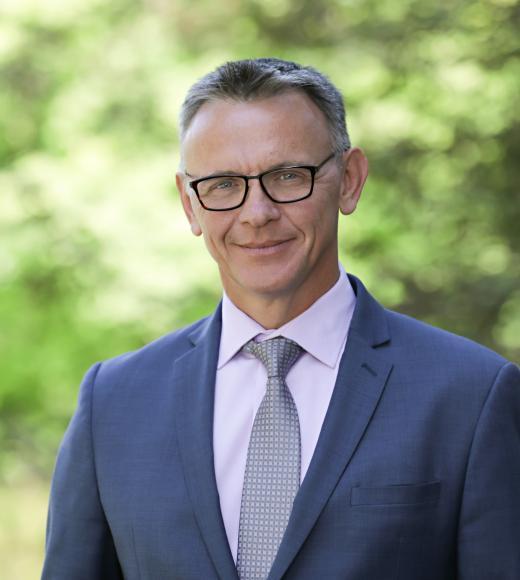
In a paper published last week by Physical Review Letters, Jean-Pierre Delplanque, a professor in the Department of Mechanical and Aerospace Engineering and the dean of graduate studies at the University of California, Davis, and a team of researchers from Lawrence Livermore National Laboratory (LLNL) and Sandia National Laboratories, have developed a scaling law to analyze the kinetics of high-pressure, rapid solidification of metastable liquids observed in national laboratory and academic experiments over the past few decades.
Scaling laws are like a secret code used across science to break down complex phenomena into simple mathematical expressions. These equations help us to understand how one factor in a system relates to other factors that determine the system's behavior. For example, Kleiber's Law, one of the best-known scaling laws, observes that metabolic rates of many organisms — from mice to elephants, and humans even — scale with body mass to the ¾-th power. This simplicity hints at an elegant underlying law in the phenomena being studied.
The temperature and pressure conditions under which phase transitions such as solidification occur are given by "maps" called phase diagrams. These maps apply only under equilibrium conditions, meaning that the transition is given enough time to fully occur. The experiments analyzed by the team, however, use a dynamic-compression platform to rapidly compress liquids in just tens to thousands of nanoseconds. This high-speed compression allowed them to explore exotic liquid states that solidify at pressures that lie far beyond what phase diagrams predict for equilibrium conditions.
Through a combination of experiments, theory and computational simulations, the team has derived a scaling law that explains and quantifies this compression-rate dependence across different experimental platforms. The scaling law is a culmination of several years of both theoretical and experimental study across a variety of systems; the published modern experimental data on solidification under dynamic compression, most of which pertain to water; and the team's own experiments conducted on gallium at the Thor pulsed-power machine at Sandia.
With further testing and refinement, the new scaling law may allow researchers to predict rapid solidification in various materials without needing experiments or simulations. It also invites important questions, like whether similar scaling patterns apply to other high-pressure phenomena, such as solid-to-solid changes or melting under extreme compression. In their paper, the team also highlighted an interesting application — using the scaling law to determine the melt temperature of materials through dynamic-compression experiments.
Read the full article from Lawrence Livermore National Laboratory